The year 2020 marks the end of a decade of unrest for New Zealand. The global coronavirus pandemic might be the first challenge that springs to mind, but it is only the most recent in a series of major disruptions. For much of the decade we’ve also been dealing with the ground literally moving under our feet. September 4th is the 10th anniversary of the magnitude 7.1 Darfield earthquake that kicked off the Canterbury Earthquake Sequence and a decade of seismic activity that has impacted lives and livelihoods of many New Zealanders. Here we summarise a few of the many geoscience and engineering advances that are helping New Zealand become more resilient to natural hazards.
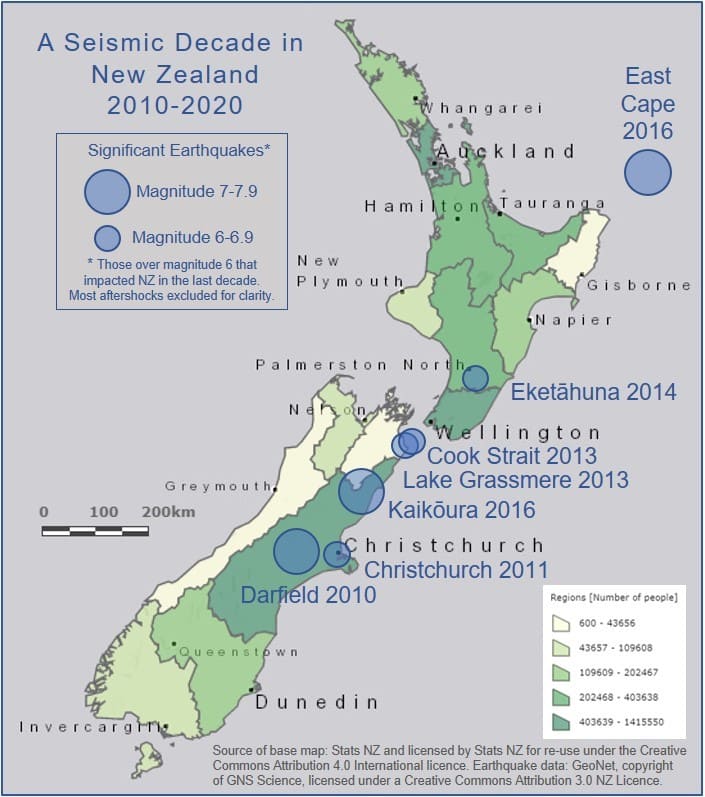
As surely as the sun rises every day, the crust of the Earth has been doing its usual work of deforming in response to movement of tectonic plates. Recently in the New Zealand region, it has been a little busier than usual and there have been a few more magnitude >7 earthquakes than expected when compared with the long-term average. Prior to 2010, many of the large earthquakes occurred in remote places such as Fiordland where they had little impact on people. The particular challenge of the last decade is that most of the large earthquakes happened close to towns and cities and at very shallow depths.
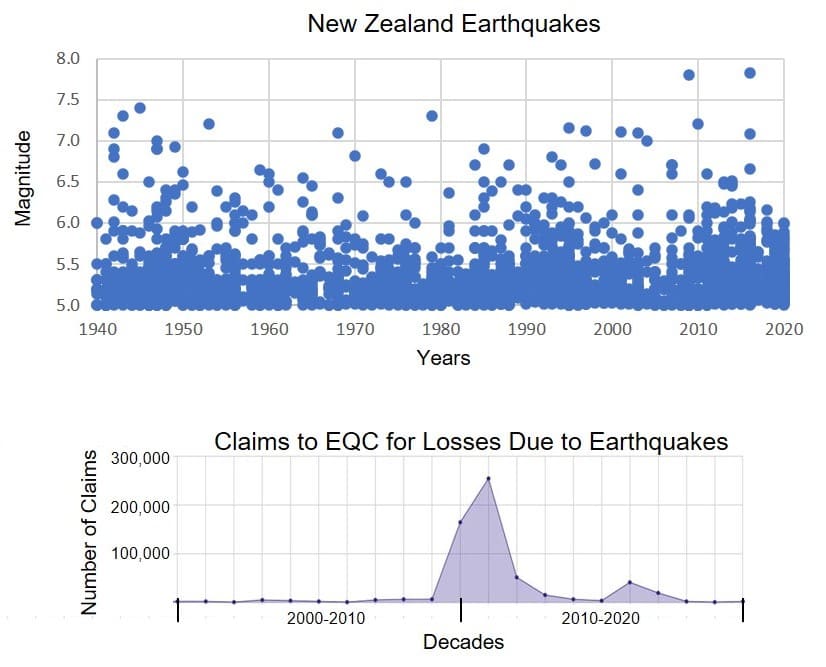
The Earthquakes
The purpose of remembering these earthquakes individually is not to re-traumatise ourselves but to reflect in awe at what we’ve come through as a country. Each significant earthquake of the last decade was very different in character and impact, reminding us of the diversity of physical processes that shape New Zealand. Like a harsh teacher, each earthquake taught us new things and handed out different challenges for our businesses, communities, economists, emergency responders, engineers, families, farmers, insurance providers, lawyers, leaders, media, medics, policy makers, politicians and scientists. Together, over the decade, we have climbed steep learning curves in many areas. Recovery has been a marathon effort. What matters now is how we take what we’ve learnt into the future.
Ten years ago, early in the morning of Saturday 4th September in 2010, Canterbury was struck by the magnitude 7.1 Darfield Earthquake. Earthquakes of this magnitude occur several times each decade on average but this was the first magnitude >7 earthquake to affect a highly populated part of New Zealand since the 1931 Hawke’s Bay earthquake. The earthquake started 10 km beneath the Canterbury plains (that’s a shallow earthquake) and broke the crust in numerous places reaching the ground surface along 30 km of the Greendale Fault. Ground shaking near the epicentre was the highest ever recorded in New Zealand at 1.25 times the acceleration due to gravity. There was widespread liquefaction and spreading of the ground adjacent to waterways in eastern Christchurch and Kaiapoi, where a few homes were torn apart. There was extensive damage to some older unreinforced masonry buildings, but many more buildings performed well and there were no deaths and few major injuries. Services were disrupted for a time but were rapidly restored. The broad consensus was, that for a major earthquake occurring within 40 kilometres of a city, the performance of buildings and infrastructure was generally good or as to be expected. Before long, the mood was to ‘get on with the recovery and return to normal’. However, this event was only the beginning of a series of earthquakes that became known as the Canterbury Earthquake Sequence.
If you’re looking for earthquakes close to cities, it would be hard to find one anywhere in the world closer than the magnitude 6.2 Christchurch earthquake of Tuesday 22nd February 2011. It hit the bullseye of the Christchurch Business District with the epicentre located only 5 km away on the outskirts of the city and the hypocentre at the very shallow depth of 5 km. In the Port Hills the ground moved vertically twice the force of gravity, flinging objects into the air and blowing the previous record, from the Darfield earthquake, out of the water. Shaking in the CBD was greater than that used in structural design standards for New Zealand buildings. The arrow of time was also cruel: the earthquake struck at lunchtime on a weekday. The consequences were horrific. One hundred and eighty-five people died – mostly in two collapsed buildings – and thousands were injured, some grievously.
This earthquake occurred on faults buried beneath the city that did not rupture to the ground surface. Therefore, there was no damage from surface rupture and no tsunami but all other earthquake hazards – ground shaking, subsidence (increased flood risk), lateral spread, liquefaction, uplift, rockfall – presented major issues for different suburbs of the city. Building damage in the central city was so extreme that a large part had to be cordoned off. Liquefaction and lateral spread were so extensive that many roads were impassable, cars were buried in silt, houses sunk into river banks, underground tanks and pipes ‘floated’ to the surface. Much of the network of underground pipes in Eastern Christchurch, having been shaken about in a basin of mud by the earthquake, was extensively damaged – the cargo of precious drinking water and sewerage now randomly mixed and no longer reaching intended destinations. Rockfall made access to southern coastal suburbs dangerous and many houses unliveable. Reinstating everything needed for community life seemed untenable, in terms of both time and cost, which was either prohibitive or, worse still, unknown. In the end, in the worst affected areas, a red zone was defined in which rebuilding was considered impractical.
Aftershocks are harsh. Although they generally decrease in magnitude and frequency following a mainshock, aftershocks occur in an area already shaken by a larger earthquake. Aftershocks may be smaller but they act on land already loosened, buildings already weakened, hearts and minds already stretched to the limit. To add to the psychological challenge, the aftershock sequence in Canterbury was dense and prolonged. Aftershocks were an almost constant backdrop to recovery efforts. Thousands were felt and even more were recorded on seismographs. These were not only psychologically unsettling but they caused further damage numerous times through the 2010-2016 Canterbury Earthquake Sequence. Major additional damage occurred in the magnitude 6.0 aftershocks of June 2011 and December 2011. By the time of the 2016 Valentine’s Day aftershock, there was reasonable confidence that the rebuilding of Christchurch was on the right track. In this magnitude 5.7 earthquake, which occurred offshore near New Brighton, there was moderate liquefaction nearby but relatively little damage. Lessons learned had been applied to the repairs and they performed well in this test.
Before anyone involved in earthquake response had had so much as a weekend sleep-in, the Cook Strait earthquakes occurred. These included the magnitude 6.5 Cook Strait earthquake on Sunday 21st July 2013 and the magnitude 6.6 Lake Grassmere earthquake on Friday 16th August 2013 as well as a number of large foreshocks and aftershocks. These earthquakes caused significant damage in Seddon and other communities in north-eastern Marlborough. Wellington was also moderately shaken, which perhaps brought home to the capital city, the magnitude of what Christchurch was still going through.
In 2014, the magnitude 6.2 Eketahuna earthquake of Monday 20th January, extended the experience of ground shaking further north. It was widely felt in both islands but was deeper than the Cook Strait earthquakes so damage was much less.
The magnitude 7.1 East Cape earthquake occurred on Friday 2nd September 2016 and was widely felt. Thankfully, it was too far offshore (125 km northeast of Te Araroa) to cause much damage. A small tsunami was generated which reminded us of the potential for large, local tsunamis arriving on our shores without warning.
The grand finale to our decade of seismic unrest was a sleep-defying two minutes of shaking for central New Zealand at two minutes past midnight on Monday 14th November 2016. Early on, the magnitude 7.8 Kaikōura earthquake caused confusion. As data streamed into GeoNet from seismographs, strong motion instruments, and tide gauges, the estimated magnitude increased, the apparent depth decreased, large aftershocks added to the chaos, the tsunami threat appeared, disappeared, and appeared again, all in the darkness of a November night. Once enough time had passed to analyse the instrumental data and to make observations on the ground, it became clear why this earthquake was so hard to read: it was big and complicated. Ground shaking broke records yet again with a recording of three times the acceleration due to gravity made near the epicentre. In two minutes, the release of seismic energy travelled over 150 km – starting near Waiau in North Canterbury, then dutifully following State Highway 1 and the Main North Railway north to Blenheim in Marlborough. On its journey up the coast it broke the ground surface on numerous faults, raised seabed out of the water, shook steep hillsides to collapse, blocked rivers, and triggered a tsunami.
There were two deaths. The timing of the earthquake was fortunate as most people were safely in bed, not out on the roads in the path of high-speed boulders or sitting in offices beneath vulnerable suspended ceilings. The worst affected areas along the fault ruptures were the farming towns of North Canterbury and Marlborough, small coastal settlements, and the town of Kaikōura. The direction and amount of seismic energy release meant that Blenheim and Wellington were also affected. Destruction of critical infrastructure was one of the biggest challenges posed by this earthquake. The main coastal transport route in the northern South Island was decimated – in places the road and railway had disappeared completely under mountainsides that had fallen to the sea. In other places rail tracks were buckled up with curves and twists like a new form of roller coaster, or they hung useless in the air, or were strewn across the beach, and everywhere rents, ruptures, fissures, cracks, crevasses, marked the land. This left Kaikōura and many smaller settlements cut off by land from the rest of the country. Rebuilding nationally important infrastructure was a major focus of the recovery.
Science Advances
Loss of life due to building collapse in the Christchurch earthquake is the biggest tragedy of the seismic decade. Massive social disruption, economic loss and decline in wellbeing followed quickly. Damage to natural and built environments has taken, and is still taking, mammoth efforts to repair. The earthquakes of the last decade have challenged us in numerous ways. However, there’s nothing like disaster to act as impetus for improvement. On the occasion of this Darfield earthquake anniversary, we revisit some of the science that has been a crucial part of the disaster response and is continuing to shape pathways to better and safer lives. Science also contributes to ongoing scrutiny to ensure that future risk is minimised and managed as cleverly as possible. As we are currently witnessing in 2020 with the arrival of a new and virulent virus, sound background science combined with a nimble science response, improves our ability to manage crises appropriately and keep people safe. Continued scientific effort will be essential in mitigating future risk.
Seismology to the Fore
Seismologists are at the forefront of the science response to earthquakes. Within minutes of an earthquake occurring, they review data from seismographs and tell us where it is centred, how deep it is, and how big it is – essential information for launching a response. Seismological information is at the core of decision making from the moment the earthquake occurs. Over the decade seismologists have quantified thousands of earthquakes to help with the national response to the damaging events. They have documented record-breaking statistics – not just once, but in three successive earthquakes strong motion instruments recorded the highest ever groundshaking in New Zealand. These observations explained why, in places, the shaking felt so violent and did so much damage. Measuring these record-breaking accelerations has, in part, been due to having denser measuring networks with instruments in the right place at the right time. In response to issues encountered immediately after the Kaikōura earthquake, strong motion seismic data is now streaming at a faster rate than previously, to speed up its availability for analysing large earthquakes and their impacts on buildings and infrastructure. Seismologists, like most scientists, are creative at heart so they’ve found ways of using the raw data of earthquakes to discover new features of how the crust suddenly breaks and what geophysical repercussions there are. From models of the movement that happened on the actual fault plane, animations showing the path of seismic energy release, and simulations of ground motion, we are not only better able to understand the very disconcerting felt experience of earthquakes, but also what to expect from future events.
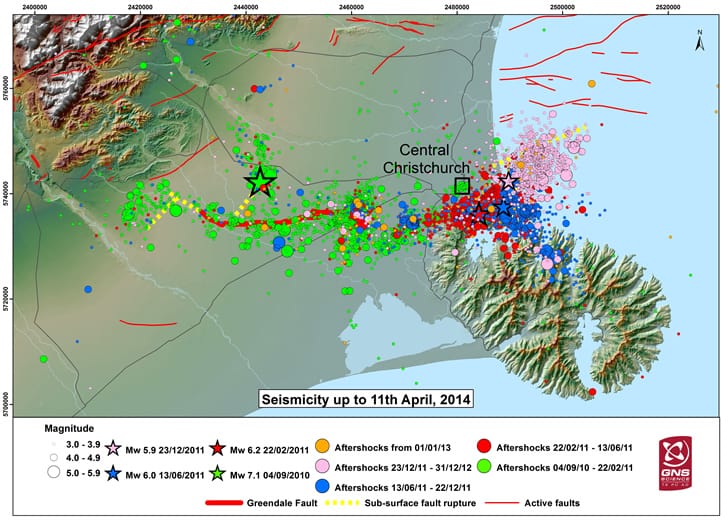
GeoNet: The Engine Room
The vehicle behind this all-important seismological information is GeoNet, New Zealand’s geological hazard monitoring system. GeoNet operates an extensive network of geophysical instruments and collects, analyses and communicates the data on earthquakes, volcanoes, tsunamis and landslides. GeoNet is a shining example of a collaborative initiative that was up and running in time for the seismic decade and evolved rapidly to meet what was needed from it. At the time of the Christchurch earthquakes, GeoNet transformed from a quiet corridor of technicians and scientists doing fairly invisible work to an energetic hub, collecting and disseminating information that half the country was relying on or using as a form of stability in a time of crisis. Ken Gledhill, then Director of GeoNet, said of those times, “I learned that science can sometimes comfort as well as inform.”
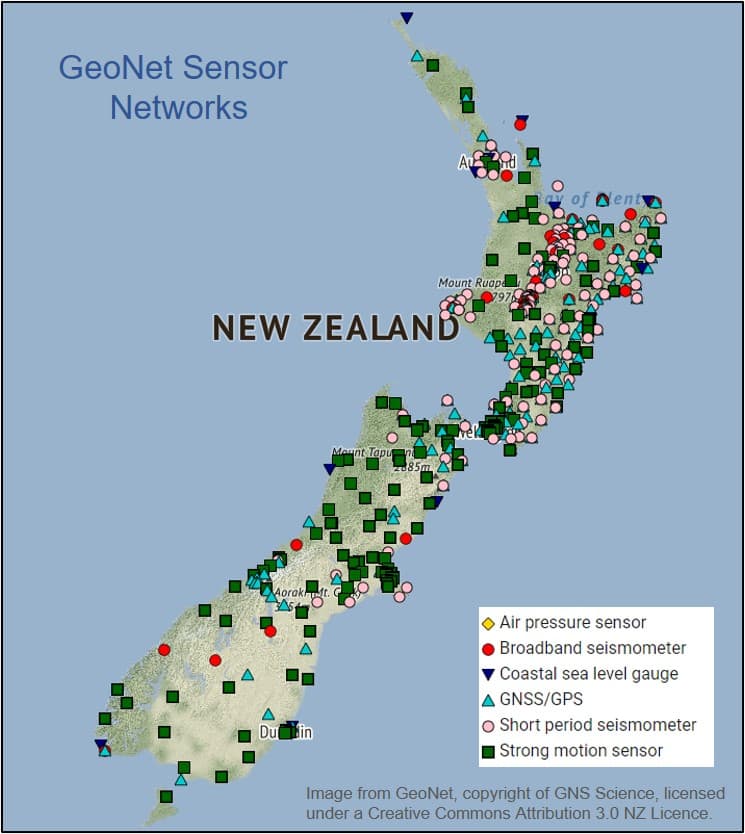
Early on in the decade, GeoNet stepped up in its role of science communication. It had ready availability of duty scientists to discuss events via various media channels, and it had mechanisms to quickly web-publish information such as aftershock probabilities. In addition, GeoNet provided a means for scientists to provide their latest findings to decision makers and the public. Over the decade, GeoNet became well-practised at response planning and providing advice to the Department of the Prime Minister and Cabinet, the Prime Minister’s Chief Science Advisor and the National Emergency Management Agency. Following the Kaikōura earthquake, in which the duty seismologist, tsunami experts’ panel, and rest of the GeoNet response team, had to conduct the first hours of response from their homes, having been shaken awake by the earthquake, there were strong calls for a 24-7 monitoring capability. This has now come to fruition with the National Geohazards Monitoring Centre opening in 2018.
Watching our Waters
It’s been a long time coming, so scientists are celebrating the fact that a network of buoys are currently being installed in local waters to help with tsunami detection. A network of DART buoys (Deep-ocean assessment and reporting of tsunamis) around the Pacific Ocean is already in place and is used by the Pacific Tsunami Warning Centre to provide tsunami warnings for all affected countries. This is great for tsunamis coming to New Zealand from other parts of the world. However, when earthquakes occur close to our shores, we have had no instrumental method for telling us whether or not a tsunami is on its way. The East Cape earthquake of 2016 graphically illustrated the need for tsunami warning buoys closer to New Zealand. Installation of an array especially designed for New Zealand, that will also help our Pacific neighbours, is currently underway.
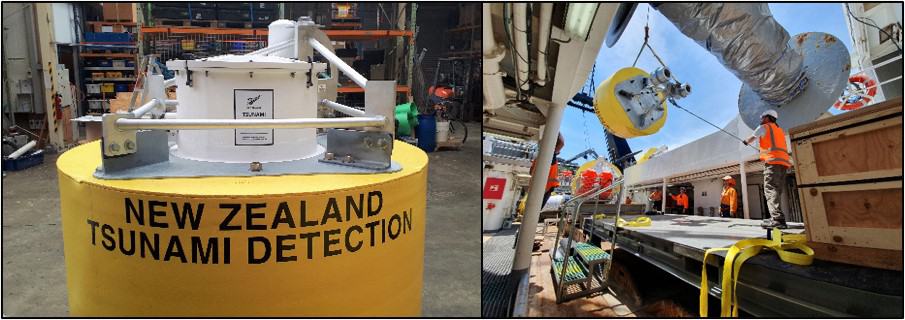
Measuring Earth from Space
Amongst its suite of technology, GeoNet has a network of around 200 Global Navigation Satellite System (GNSS) receiving stations throughout New Zealand. These are high-end versions of the GPS-based location systems in smart phones and cars. Changes in the positions of the stations relative to each other can be measured to millimetre accuracy. As New Zealand slowly deforms due to tectonic forces, or suddenly during earthquakes, these movements are tracked by the GeoNet GNSS network. Combined with other Earth sensing data from international satellites, these measurements can be used to map fault movement, and, after the Darfield and Kaikōura earthquakes, they allowed the complex rupture processes to be accurately determined. We now know much more about how our plate boundary works during big earthquakes. This helps us interpret what we see preserved in the long-term geological record which is vital because our written record of earth movements in New Zealand is short – less than 200 years.
Relieving Stress Slowly
An important discovery soon after the first GNSS stations (previously called GPS stations) were established in New Zealand more than 15 years ago, was that the usual slow movement of the Earth’s surface caused by tectonic plate motions periodically speeds up and reverses for weeks or months at a time. This slow-slip happens on the interface between the two major tectonic plates. It transfers built-up stress in the crust without the damaging rapid movement of large earthquakes. Slow-slip events have been observed repeatedly beneath parts of the North Island, including immediately following large earthquakes. The most widespread triggering of slow-slip events following a crustal earthquake anywhere in the world was measured in New Zealand following the Kaikōura earthquake. With this movement occurring beneath Marlborough, Gisborne, Pōrangahau and Kāpiti, the question arose: was the Hikurangi subduction zone about to rupture in a large earthquake? The relationship between large earthquakes and slow-slip remains a significant scientific challenge but at least we are at the stage of observing slow-slip events – we have our finger on the pulse of New Zealand’s biggest fault.
Geophysical Solutions
The most pressing geoscience question of all the earthquake recoveries over the decade was whether there would be another large earthquake within the recovery period. Ever-willing to respond to demand, Kiwi geophysicists set about figuring out how to answer this question rigorously. Aftershock forecasts were provided frequently via GeoNet. Following the Kaikōura earthquake, an earthquake hazard model specific to the region was developed. For the first time ever, the likelihood of slow-slip events triggering a subduction zone earthquake was incorporated into the model. The Kaikōura earthquake hazard model enabled well-informed decisions to be made by everyone from infrastructure teams working under unstable hillsides to politicians managing safety and guiding the pace of recovery.
Geological Ground-truthing
Measurements from space are an exciting technological advance, but they are not a complete replacement for being on the ground to observe what has happened. Geologists are trained to get their data from the land so you would have been hard pressed to find any in the office after these earthquakes. Their first role in an earthquake response is to look for evidence of faults having ruptured to the ground surface and land that has shifted vertically or horizontally. After each earthquake, detailed mapping and measuring of these features provided realistic inputs for the modelling of what had happened below the surface and development of a picture of what land had moved and by how much. Following the Kaikōura earthquake, after spending more time in the field than ever before, with the take-over of motels to become field camps, and national coordination of local personnel and helpers from overseas, as well as some offshore surveying, earthquake geologists mapped 24 faults that had ruptured to the ground surface in the earthquake and 100 km of coastline that had moved vertically. Thanks largely to this fieldwork, the Kaikōura earthquake now has a global reputation for being one of the most complicated earthquakes ever recorded on Earth.
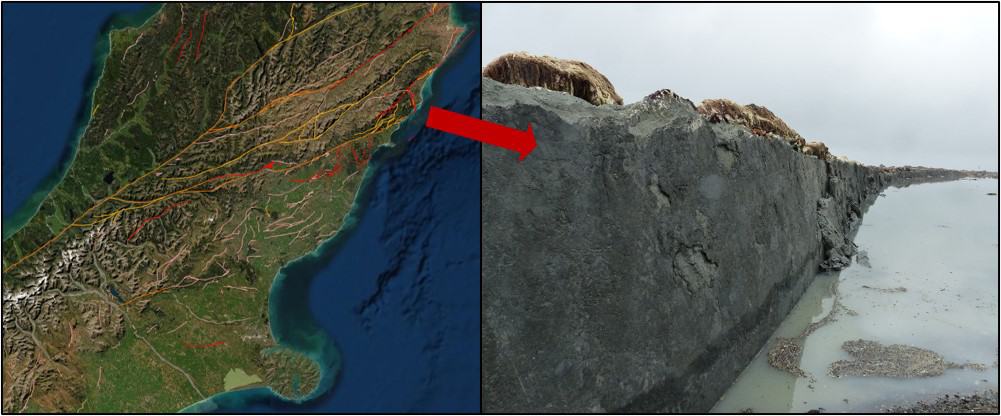
Discoveries from earthquake geologists over the decade have also shown us that our largest faults – the Alpine Fault and Hikurangi Subduction Zone – are ‘primed’ for release. The geological evidence for numerous past earthquakes on these faults, shows us that future large to great earthquakes (magnitude 7-8) are inevitable. Such information has spurred initiatives like AF8 and East Coast LAB which are collaborations between scientists, communities and civil defence to reduce the impact of future earthquakes and be better prepared when they happen.
Surveying for Survival
At the time of the 1931 Napier earthquake, measuring how the land had moved involved time-consuming, methodical use of a theodolite and levelling staff. Surveying techniques have come a long way since then and can do seemingly magical things like seeing through vegetation. For example, the increasing coverage of LIDAR (a remote sensing survey method) in New Zealand is a real game-changer when it comes to earthquake response. From September 2010, and following each major earthquake, the Earthquake Commission (EQC) and Land Information New Zealand (LINZ) acquired aerial photography and LIDAR surveys, to guide and augment ground surveys of land movement. In areas where there was pre-earthquake LIDAR, the difference between the pre- and post-earthquake surveys showed the relative vertical movement of land. Such visualisation enabled rapid decision-making about flood risk, water supply, transport corridors and drainage – the lifelines that make towns and cities liveable.
Understanding Land
In late 2011, as a result of the Christchurch earthquake, the requirement for deep geotechnical investigations was introduced for the most vulnerable land and, recognising that geotechnical resources were scarce, an area-wide collaborative investigation programme was proposed early the following year. This was accompanied by development of the Canterbury Geotechnical Database – a repository for the extensive data collected, to establish the engineering properties of the soils and understand their performance in earthquake shaking. The database, developed for the New Zealand Government by engineering firm Tonkin + Taylor, based on GeoNet data-sharing principles, has provided a platform for geotechnical professionals to store, share and readily assess the data files of geotechnical investigations (bore holes, cone penetration tests, piezometers and groundwater monitoring, and other relevant data) during the rebuild. It now contains over 200,000 records, in the order of $350 million worth of physical site investigations, not including the additional enabling costs incurred in its acquisition. The re-use of data means that future collection can be targeted, thereby reducing costs while improving shared understanding of risk over time. The Ministry of Business, Innovation and Employment (MBIE) and EQC have taken over stewardship of the database and data can be entered and accessed for anywhere in New Zealand. This nationwide resource is enabling a much greater understanding of subsurface conditions so that transport, building projects, and risk modelling is much better informed.
Engineering Stronger Land
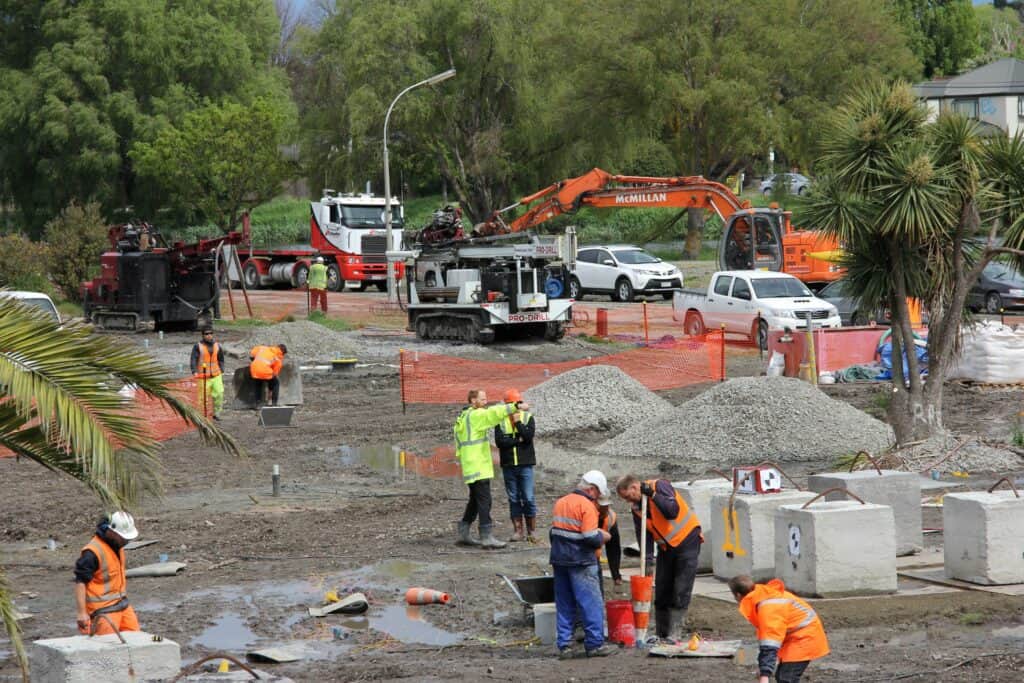
Some of the post-earthquake learning has been truly global. For example, eastern Christchurch and Kaiapoi experienced liquefaction from the Darfield earthquake on a scale not previously seen in a residential setting. New tools to assess and communicate liquefaction risks have emerged from intensive collaborative research, including experimentation and commercial development of ground improvement methods that are viable at a suburban scale. This work involved one of the largest teams of geotechnical engineers and scientists ever to work on a single project in New Zealand. The work has led to substantial advances in the global understanding of liquefaction and its assessment and mitigation. The Ground Improvement Programme (GIP) identified, developed, and trialled, practical and affordable shallow ground improvement methods that could mitigate the potential for liquefaction and associated damage to houses built on land vulnerable to liquefaction. Subsequent changes to regulatory guidance have provided more affordable ground improvement options for building or rebuilding houses on residential land. Training courses for urban planners and engineers have been delivered in all regions where land is susceptible to liquefaction. The Stronger Christchurch Infrastructure Rebuild Team (SCIRT) had the almighty task of rebuilding Christchurch’s roads and underground water and wastewater networks. Crucial to this work was understanding ground conditions and using innovative engineering solutions to overcome the challenges presented by earthquakedamaged land. It is testament to this organisation that it won, among many awards, the Brunel Medal for excellence in civil engineering in 2013 from the Institution of Civil Engineers in the United Kingdom.
Landslide Taming
Landslides are not easy to tame, but for the landslides threatening buildings or infrastructure, engineering geologists used a wide range of monitoring technology to keep a close eye on them. There were over 20,000 landslides as a result of the Kaikōura earthquake. Some had to be moved off roads and railways but many were far too big to tackle and instead had to be built around and controlled. Helicopters with sluicing buckets removing loose material, and abseilers with explosives to blow up big boulders, became a normal part of the recovery works along State Highway 1 and the Main North Railway. In this corridor the scars of giant landslides remain partially concealed behind huge rock walls and rockfall canopies. Where landslides dammed rivers, constant monitoring was undertaken to ensure there would be adequate warning of any sudden dam burst. ongoing research into the location and volume of debris working its way down from the mountains to the coast will help us work out how to build resilience into our transport networks.
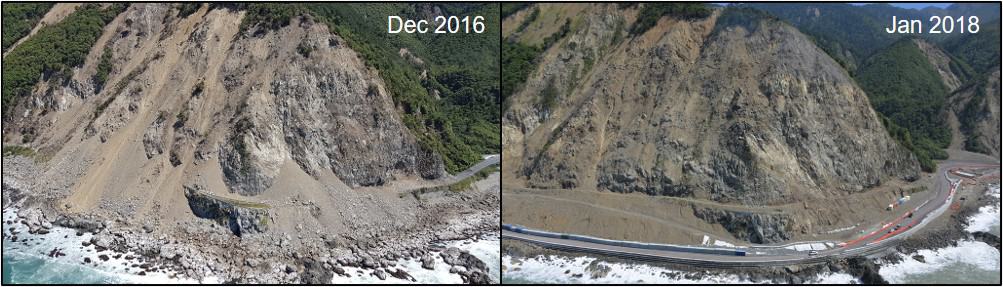
As if there wasn’t enough chaos from landslides onshore, the potential for landslides out in the ocean was surveyed after the Kaikōura earthquake because of their ability to trigger tsunamis. Scientists monitoring the offshore Kaikōura canyon found that the earthquake had triggered enormous amounts of sediment to flow down the canyon and along the deep sea Hikurangi channel for hundreds of kilometres. The high-energy currents stripped the seabed of life but did not pose any risk to life onshore.
Engineering Innovations
Engineers will tell you there’s an engineering solution for everything and they’re probably right – as long as no shortcuts are taken. The tragic loss of life and terrible injuries that occurred in the collapse or partial collapse of several buildings in February 2011, and a few near misses in the Darfield and Kaikōura earthquakes, highlighted the importance of rigorous design, consenting and construction practices. Most buildings performed well in the earthquakes in terms of life safety – many even exceeding the demands for which they were designed, in some cases decades before the current structural codes were enacted. But we now understand there are vulnerabilities in some modern buildings. These can occur where the architectural designs have departed from regular forms – for example, some homes built on hillsides across split levels, where the building responds unevenly to shaking. This effect is just as relevant in larger buildings with stiffness incompatibilities between stairs, floors and frames resulting in costly damage and uncertainty about repairs and future performance. We have seen how business recovery and livelihoods can be profoundly affected by decisions on whether, or not, a home or workplace can be re-occupied and when. Out of these experiences comes a suite of approaches that will enable buildings to be used immediately postearthquake as well as to save lives. For example, formal guidance now exists for the safe incorporation of nonstructural elements into buildings such as cladding, ducting, pipework, suspended ceilings, and electrical equipment. The protection of existing buildings with solutions such as viscous dampers, base isolators, and extrusion dampers was not a feature of Christchurch buildings prior to the earthquakes and is now being used extensively in the city, with more being commissioned further afield. The strengthening of unreinforced masonry buildings has also come a long way with the use of seismic gaps to allow movement, plywood diaphragms, concrete ring beams and glass-reinforced polymer to strengthen brickwork. In addition, the removal of heavy chimneys, awnings, and stone facades has received increased focus following deaths and injuries from these features in the Christchurch earthquake.
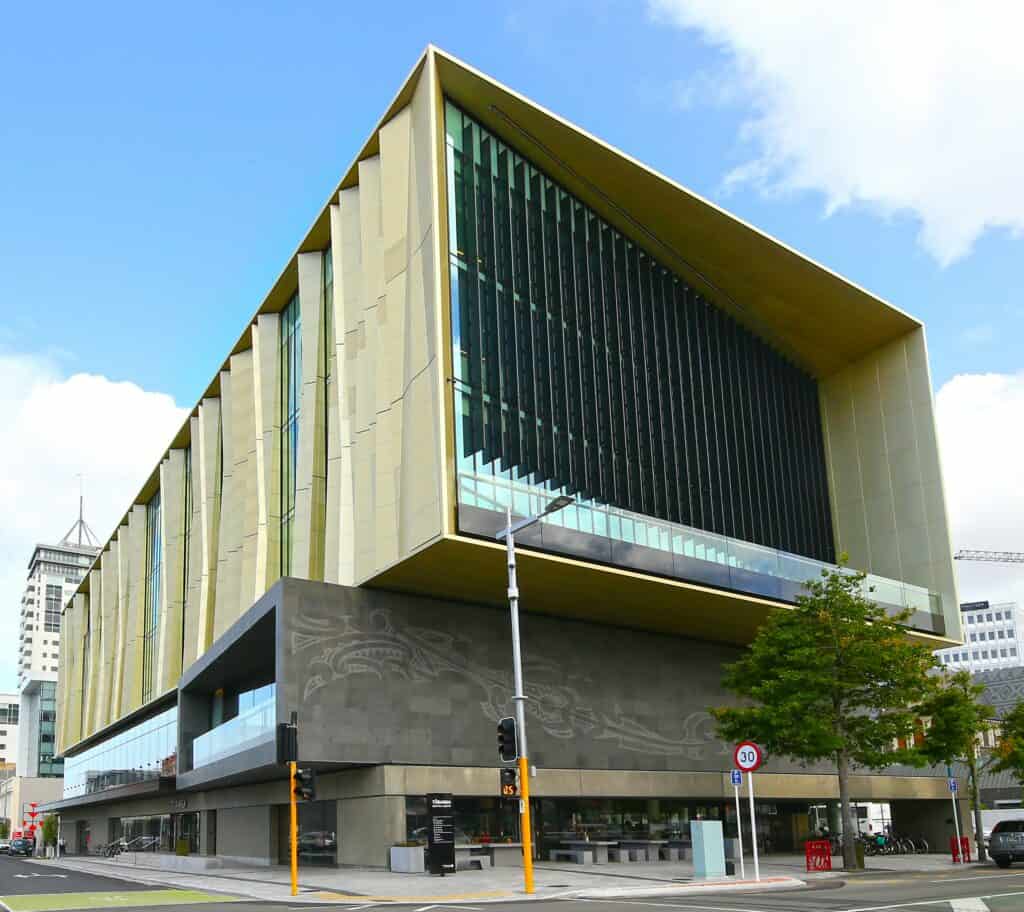
Citizen Science
Important contributions to the science response of all these earthquakes came from the people of New Zealand. Hundreds of thousands of citizens submitted earthquake felt reports numerous times over the last decade and these provided a visually striking, immediate, and useful indication of where and how strongly each earthquake had been felt. In addition, observations of earthquake impacts, and demands for solutions from communities and individuals, are driving the scientific process all the time. Finally, the experience of this seismic decade has no doubt improved individual awareness and raised levels of preparedness across the country.
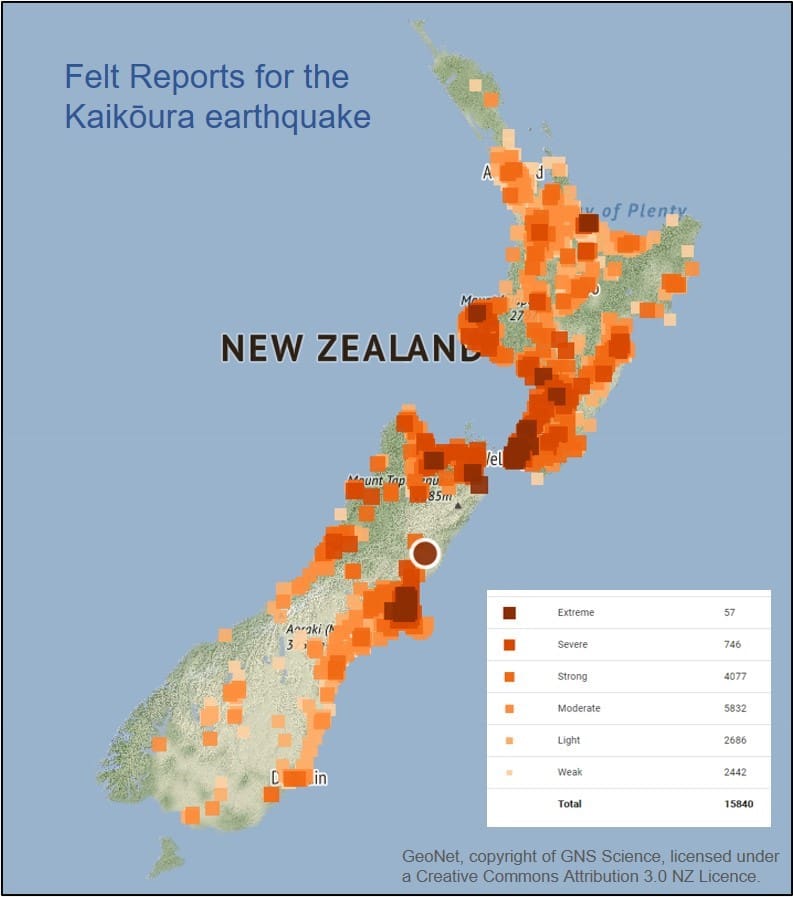
Conclusion
Disruption, a shake-up of the status quo, has a reputation for being a spring-board for new ideas, an accelerant for innovation, and a harsh light shining on what we could have done better. The disruption caused by New Zealand’s decade of earthquakes has been no exception. New Zealanders adapted quickly to living in tents, sharing portaloos, collecting water, cooking food for the displaced, sharing resources and, out of apparent chaos, came brilliant solutions. It was no different in geoscience – the earthquake response led to internationally significant discoveries about how the earth works, new knowledge on the ‘where, what, and why’ of earthquakes, innovation into new ways of doing things, clever use of technology, extensive collaboration and development of new resources. These advances mean we understand more about earthquake hazards, we are better prepared, but we are only just starting to lower the risk we face from future events. We have by no means maximised the knowledge we have gained. So far, much of the risk reduction has occurred in Christchurch alone, through redzoning land or improving it and using improved engineering controls for the rebuild. There is much work to be done to apply higher standards nationally (see part two in this series). And in light of what we now know about the readiness of the Alpine Fault and the Hikurangi Subduction Zone to rupture in large earthquakes, there is no room for complacency.
About the Authors:
Kelvin Berryman established Berryman Research and Consulting in 2020 after 45 years with GNS Science where he undertook hazard and risk research in earthquakes and other natural hazards and managed major research initiatives. He was awarded a QSO in 2011 for services to science and the Canterbury earthquake recovery. He is a Fellow and Life Member of the NZ Society for Earthquake Engineering and he is an elected Fellow of the Royal Society of New Zealand.
Hugh Cowan is a consultant in natural hazard risk management and disaster risk financing. Hugh previously guided EQC’s reinsurance programme and its research investments for more than a decade. He earlier led the establishment of New Zealand’s geological hazard monitoring system, “GeoNet” at GNS Science. Hugh has contributed to international disaster risk financing and infrastructure development in Asia, the Americas and
Scandinavia. He is a Fellow and Life Member of the NZ Society for Earthquake Engineering.
Ursula Cochran is a science writer based in Wellington. She has a particular interest in earthquakes having worked as an Earthquake Geologist at GNS Science for 18 years.